Photo credit Jennifer Uppendahl on Unsplash
Wild Carbon: A Synthesis of Recent Findings on Carbon Storage in Old Forests
Communication & Education
December 2021 | Volume 27, Number 3
We find ourselves not at the edge of a precipice, but beyond it. “Climate change” was once a warning bell suggesting a dire future but is now a full-fledged alarm signaling direct and immediate impacts on human and other-than-human communities. With heat waves, droughts, wildfires, melting glaciers, and record-breaking storms making headlines weekly, if not daily, the effects on people and society are unfolding in real time. While our wild relatives make the news less frequently, the consequences of climate chaos on their lives is no less devastating or widespread – and indeed, their well-being is indivisible from our own.
All living beings will experience the hardships of a hotter, more volatile climate no matter how quickly we act to reduce our collective carbon footprint. Yet this fact does not nullify our collective responsibility to do all we can to make the best possible choices, informed by current research, to employ our last best chance of reducing atmospheric carbon dioxide – currently reported at an average of 409.9 parts per million by the National Oceanic and Atmospheric Administration – to safe levels for nature and people. And while technological inventions to capture carbon have been the subject of popular attention and hope of late, it turns out that the most economic and effective systems to sequester and store carbon were “invented” long before humans walked the Earth. They are called ecosystems.
Forests, grasslands, oceans, and wetlands are considered “natural climate solutions.” These ecosystems are already doing the work that carbon capture technology hopes to achieve – at a fraction of the cost and with innumerable cobenefits to wildlife and people. With deforestation eating away at the few intact natural places left on Earth, meaningful legal protection – coupled with rewilding and restoring degraded land – is necessary if these wondrous places are to continue doing their important work while also providing refuge to diverse, declining species and supporting the health of human communities. Initiatives such as 30×30 (www.campaignfornature.org), Nature Needs Half (www.natureneedshalf.org), and the UN Decade on Ecosystem Restoration (www.decadeonrestoration.org) are indicative of a global shift in consciousness toward protecting nature as an essential solution to climate change, and a critical ingredient for a thriving, healthy planet.
An emphatic embrace of natural climate solutions to mitigate global overheating does not require an either/or choice between managed and unmanaged forests. However, conserving unmanaged wild forests is a proven, scalable, and cost-effective strategy that is complementary to, not exclusive of, the continued conservation and stewardship of well-managed woodlands.
– Jon Leibowitz, Executive Director of Northeast Wilderness Trust
Wild Carbon
Wild nature has a right to exist simply for its intrinsic value and rich biodiversity, but recent peer-reviewed research is shedding light on an added benefit that old unmanaged forests offer exceptional carbon storage capacity. A long-standing debate over the value of old forests in capturing and storing carbon has prompted a surge of synthesis studies published in top science journals during the past decade. The findings of the studies summarized herein as six well-supported truths resolve this debate and demonstrate the irreplaceable value of older forests.
Trees Accumulate Carbon Over Their Entire Lifespan
Plants absorb carbon dioxide from the air and transform it into carbon-rich sugars. These are then converted to cellulose to create biomass (wood, bark, root, and leaves) or are transferred belowground to feed the root-fungal (mycorrhizal) networks. Over the long lifespan of a tree, large amounts of carbon are removed from the air and stored as biomass. Growth efficiency declines as the tree grows, but corresponding increases in the tree’s total leaf area are enough to overcome this decline and thus the whole-tree carbon accumulation rate increases with age and size. A study of 673,046 trees across six countries and 403 species found that at the extreme, a large old tree may sequester as much carbon in one year as growing an entire medium-sized tree (Stephenson et al. 2014; figure 1). At one site, large trees made up 6% of the trees but 33% of the annual forest growth. Young trees grow fast, but old trees store a disproportional amount of carbon.
Old Forests Accumulate Carbon and Contain Vast Quantities of It
Old-growth forests have traditionally been considered negligible as carbon sinks. Although individual trees have an increasing rate of carbon sequestration, the forest stands (groups of trees) have an “S-curve” of net sequestration rates. The rate of carbon accumulation in the ecosystem is slow at first, then speeds up for some time, then slows down again after many decades. The expected carbon neutrality of older stands was thought to be due to sequestration during tree growth being balanced out by carbon emissions from respiration and decomposition.
To test this, and determine if old forests are actually carbon neutral, an international team of scientists reviewed 519 published forest carbon-flux estimates from stands 15 to 800 years old. They found that contrary to expectations, 75% of stands more than 180 years old sequestered more carbon than they emitted, and the chance of an old-growth forest being carbon neutral was less than 1 in 10 (Luyssaert et al. 2008). They concluded that old-growth forests act as carbon sinks more often than not, steadily accumulating carbon as well as storing vast quantities of it. They argued that accounting rules for forest carbon markets should give credit for leaving old-growth forest intact. Goldstein et al. (2020) bolstered this argument by showing that old forest carbon is “irrecoverable,” meaning it is vulnerable to release upon land use conversion and, once lost, is not recoverable on timescales relevant to avoiding dangerous climate impacts. This is important globally, as old forests in the tropics have acted as long-term carbon sinks but are now vulnerable to edge effects, logging and thinning, or increased mortality from disturbances (Brienen et al. 2015; Qie et al. 2018).
Old Forests Accumulate Carbon in Soils
The soil-carbon balance of old-growth forests has received little attention, although it was generally accepted that soil organic carbon levels in old forests are in a steady state. In 2017, Guoyi Zhou and colleagues measured the 24-year dynamics of the soil carbon in an old-growth forest at China’s Dinghushan Biosphere Reserve. They found that the top 20 centimeters of soil accumulated atmospheric carbon at an unexpectedly high rate: over the course of the study, the concentration of organic carbon in the soil increased from about 1.4% to 2.4%, and soil carbon stock increased significantly at an average rate of 0.61 metric tons of carbon per hectare per year (Zhou, G. et al. 2006). Their result directly challenges the prevailing belief in ecosystem ecology regarding soil carbon in old-growth forests and calls for further study.
Forests Share Carbon among Tree Species
Forest trees compete for light and soil resources, and competition for resources is commonly considered the dominant tree-to-tree interaction in forests. However, recent research using stable carbon isotope labeling indicates that trees interact in more complex ways, including substantial exchange and sharing of carbon. In 2016, Tamir Klein and colleagues applied carbon isotope labeling at the canopy scale and found that carbon assimilated by a tall spruce was traded with neighboring beech, larch, and pine trees via overlapping root spheres. Aided by mycorrhizal networks, transfer between species accounted for 40% of the fine-root carbon, totaling roughly 280 kilograms per hectare per year in tree-to-tree transfer (Klein et al. 2016). In a subsequent study, Morrie et al. (2017), found that mycorrhizal soil networks become more connected and take up more carbon as forest succession progresses, even without major changes in dominant species composition.
Forest Carbon Can Help Slow Climate Change
There has been debate about the role of forests in sequestering carbon and the role of land stewardship in achieving the Paris Climate Agreement goal (United Nations 2015). In 2017, Bronson Griscom and colleagues systematically evaluated 20 conservation, restoration, and improved land management actions that increase carbon storage and/or avoid greenhouse gas emissions. They found that the maximum potential of these natural climate solutions would be almost 24 billion metric tons of carbon per year while simultaneously safeguarding food security and biodiversity. About half of this could be delivered as cost-effective contributions to the Paris Agreement, equivalent to about 30% of needed mitigation by 2030, with 63% coming from forest-related actions (figure 2). Avoided forest conversion had the highest carbon potential among the low-cost solution options (Griscom et al. 2017). New research suggests that protecting forests from being converted to other uses is the most cost-feasible option by a large margin (Busch et al. 2019), and it should receive high priority as policy in the United States (McKinley et al. 2011). An analysis of 18,507 forest plots in the Northeast found that old forests (greater than 170 years) supported the largest carbon stocks and the highest simultaneous levels of carbon storage, timber growth, and species richness (Thom et al. 2019). In addition to helping stave off climate change, old forests possess critical cobenefits including building healthy soil, cycling nutrients, mitigating pollution, purifying water, releasing oxygen, and providing habitat for wildlife.
Old Forests Have Higher Net Sequestration Rates of Carbon Than Younger, More Recently Cut Ones
While it is becoming well accepted that old forests store large amounts of carbon, there is controversy over the age at which forests sequester the most carbon annually (e.g., remove carbon from the atmosphere and fix it as biomass). The confusion arises because young trees grow faster than old trees and thus the rate of sequestration in a young forest can be very high. However, in the words of a carbon scientist friend, it is “not the rate we are interested in but the volume” (C. McDonald, personal communication, October 18, 2019). As with a bank account, it is not how fast deposits are made but how large those deposits are, and young forest deposits are limited by leaf area (the total area of leaf surface where carbon exchange takes place).
Net sequestration is the difference between how much carbon the forest absorbs from photosynthesis minus how much it emits through respiration. The former is a function of the leaf area index (LAI), the latter is a function of cell respiration in soils, roots, woody debris, trunk, branches, and leaves. The limitations of young forests in net sequestration was demonstrated in 2001 by a team of researchers at Oregon State. Led by Beverly Law, they implemented a gold-standard, labor-intensive study that measured and compared all the carbon stocks and fluxes occurring in the same forest type at two stages of development (Law et al. 2001). Specifically, they compared an old mixed-age stand (50–250 years) with a recently cut stand (17 years ago) within the same dry ponderosa pine forest in eastern Oregon (figure 3).
The study’s results showed clearly that when all fluxes were accounted for, the old forest was sequestering carbon at a rate of 28 g/m2/year, while the young forest was losing carbon at a rate of -32 g/m2/year. The loss was due to the leaf area index being too small to compensate for soil respiration. The authors concluded that it would take 20–30 years for the young forest to develop sufficient leaf area to offset respiration and become a carbon sink. Until then the forest emits carbon every year.
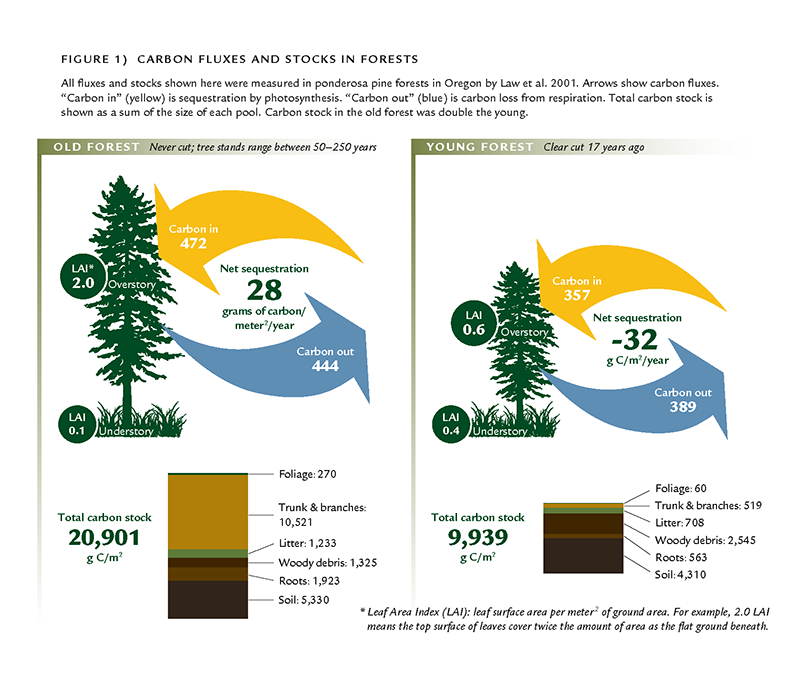
Figure 3 – Adapted from Law et al. 2001. Existing carbon stock shown in proportion to the size of the pool. Old forest carbon stock was double the young. Blue arrows show the carbon fluxes. “IN” refers to sequestration by photosynthesis. “OUT” refers to carbon loss from respiration. The difference is in bold
Similar dynamics occur in eastern forests. A carbon study of an early successional hardwood site in Massachusetts showed that the site became a net emitter of carbon immediately after harvest and was expected to return to being a sink after 10–15 years, assuming no other disturbance (Finzi et al. 2020). Eastern forests, however, show considerably larger carbon stocks and 7–10 times higher rates of carbon sequestrations than the dry forests of eastern Oregon (table 1). This may help them recover quickly where the leaf area index is sufficiently large that net primary productivity surpasses respiration.
The often-touted fast growth and high sequestration rate of young eastern forests (optimized at 30–70 years) helps the forest return to its previous state as quickly as possible, but recovery is a two-step process. First, the forest must regrow its leaf area and return to being a carbon sink (10–30 years). Second, because the forest has been losing carbon, it must make up for those losses before it reaches neutrality. If we assume it takes an equal amount of time to replace the carbon as it did to lose it, then after 20–60 years the forest may just have compensated for the early losses from respiration and will again start to sequester “new” carbon. Comparing this cycle to that of an old forest slowly and steadily sequestering carbon every year over the same time span, the old forest will remove considerably more carbon from the atmosphere despite its slower growth rate (table 1).
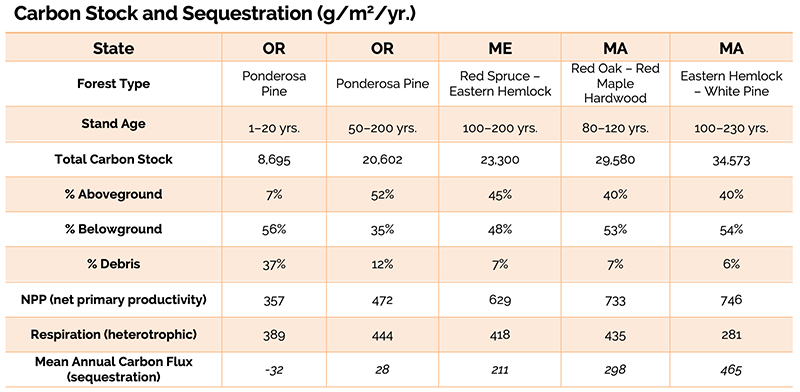
Table 1 – Comparison of Carbon Stocks and Annual Sequestration. Data sources: Law et al. 2001 (Oregon), Finzi et al. 2020 (Massachusetts), Hollinger et al 2021 (Maine).
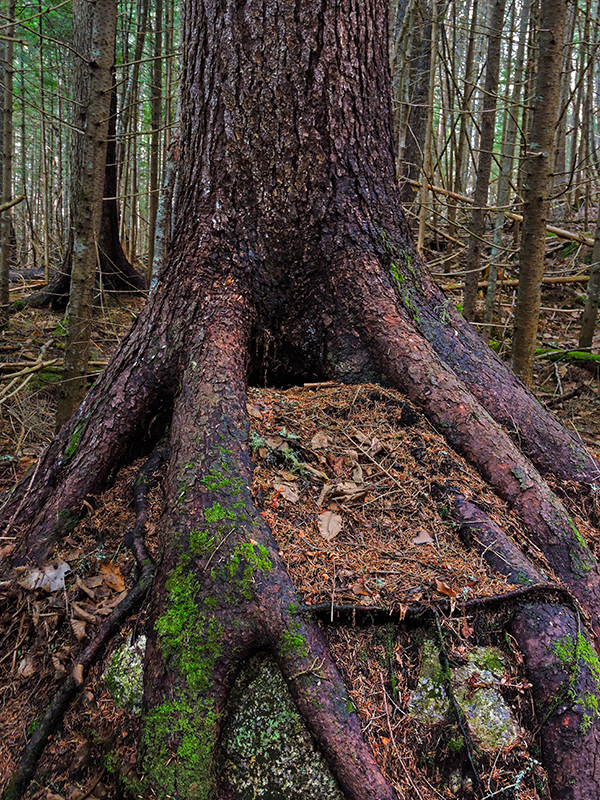
Figure 4 – Trees share carbon with one another through belowground fungal networks, and even give extra carbon to their own offspring. © Susan C. Morse.
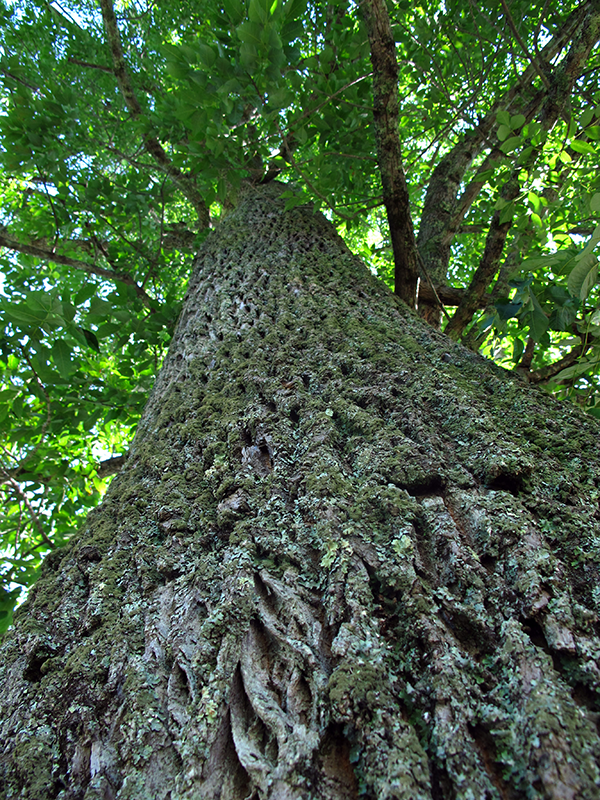
Figure 5 – This large ash tree is removing carbon from the atmosphere through its leaves and converting it to biomass (branches, wood, bark, roots) through photosynthesis. © Susan C. Morse.
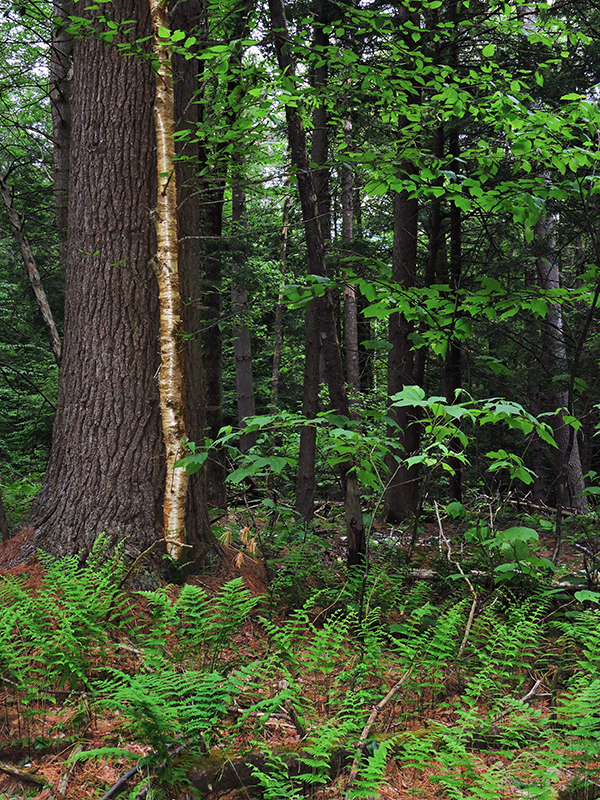
Figure 6 – The amount of carbon that would be lost from old forests is irrecoverable on a timescale relevant to preventing the worst impacts of climate change. © Susan C. Morse.
Conclusion
Recent peer-reviewed science has established that old forests are highly effective at capturing and storing carbon. It is now clear that trees accumulate carbon over their entire lifespan and that old, wild forests accumulate far more carbon than they lose, thus acting as carbon sinks. This is especially true when considering undisturbed soils only found in unmanaged forests – the role of which is yet to be fully understood. Although young forests sequester carbon at a faster rate, the volume they sequester is relatively small and insufficient to compensate for carbon losses through soil respiration, typically resulting in a net release of carbon. It takes 10–30 years for the leaf area of a young forest to expand to where sequestration offsets ecosystem respiration, and many more years for the forest to offset the losses they accrued in the first 10–30 years. Compounding evidence indicates that avoiding forest conversion is the fastest and cheapest way to sequester carbon over the next few decades and that old forest carbon is irreplaceable on timescales relevant to avoiding climate impacts.
This recent evidence challenges standing assumptions that old forests are carbon neutral (table 2), and that to achieve their maximum carbon potential forests must be managed. However, comprehending the whole story is both messier and more hopeful. It is messier because forests are subject to natural disturbances such as fires, windstorms, and insect outbreaks that affect carbon storage in ways similar to timber harvest. For example, the hemlock forest data in table 1 were collected just before an outbreak of hemlock wooly adelgid, which caused a steep decline in ecosystem productivity and flipped the forest from a carbon sink to a source (Finzi et al. 2020). But disturbances also offer hope. They rejuvenate wild forests with an influx of abundant coarse woody debris that helps mediate carbon loss, maintain root-mycorrhizal relationships, and increase biodiversity and wildlife abundance. Hope can also be found in the way that foresters are using good science to improve management practices. Retention forestry, longer rotations, and attention to soil carbon pools can promote both carbon and diversity in managed forests. Post-harvest practices that reduce carbon waste during the processing, transportation, and use of wood products is an active area of research with much promise.
Achieving meaningful carbon sequestration by 2050 requires multiple strategies: carbon-neutral forest management, extensive reforestation, and permanent protection. The latter – creating wild spaces where unmanaged forests can age and develop – should be the centerpiece of our carbon solutions.
About the Authors
MARK G. ANDERSON, PhD, is the director of conservation science for the Center for Resilient Conservation Science under The Nature Conservancy’s North America Region. He serves as board president for the Northeast Wilderness Trust.
NORTHEAST WILDERNESS TRUST conserves forever-wild landscapes for nature and people. Founded in 2002, the Wilderness Trust works across New England and eastern New York. The Wilderness Trust owns Wilderness Preserves and Sanctuaries, and protects land through legal means such as conservation easements. The organization currently safeguards more than 41,000 acres of wildlands in six states. Learn more at www.newildernesstrust.org.
Wild Carbon is the first edition of Northeast Wilderness Trust’s “Wild Works” series, which characterizes the values and benefits of wild, unmanaged landscapes.
References
References
Brienen, R. J. W. et. al. 2015. Long-term decline of the Amazon carbon sink. Nature 519: 346–347.
Busch J. et al. 2019. Potential for low-cost carbon dioxide removal through tropical reforestation. Nature Climate Change 9: 463–466.
Finzi A. C. et al. 2020. Carbon budget of the Harvard Forest Long-Term Ecological Research site: Pattern, process, and response to global change. Ecological Monographs 6.
Goldstein, A. et al. 2020. Protecting irrecoverable carbon in Earth’s ecosystems. Nature Climate Change. https://doi.org/10.1038/s41558-020-0738-8.
Griscom, B. W., J. Adams, et al. 2017. Natural climate solutions. PNAS 114(44): 11645–11650.
Hollinger, D. Y. et al. 2021. Multi-decadal carbon cycle measurements indicate resistance to external drivers of change at the Howland Forest AmeriFlux site. Biogeosciences (in review).
Klein et al. 2016. Belowground carbon trade among tall trees in a temperate forest. Science 352(6283): 342–344.
Law B. E, P. E. Thornton, J. Irvine, P. M. Anthoni, and S. Vantuyl. 2001. Carbon storage and fluxes in ponderosa pine forests at different developmental stages. Global Change Biology 7: 755–777.
Luyssaert et al. 2008. Old-growth forests as global carbon sinks. Nature 455: 213–215. doi:10.1038/nature07276.
McKinley, D. C. et al. 2011. A synthesis of current knowledge on forests and carbon storage in the United States. Ecological Applications 21(6): 1902–1924.
Morrie et al. 2017. Soil networks become more connected and take up more carbon as nature restoration progresses. Nature Communications 8: 14349.
Qie, L. et al. 2017. Long-term carbon sink in Borneo’s forests halted by drought and vulnerable to edge effects. Nature Communications 8: 1966.
Stephenson et al. 2014. Rate of tree carbon accumulation increases continuously with tree size. Nature 507: 90–93. doi:10.1038/nature12914.
Thom et al. 2019. The climate sensitivity of carbon, timber, and species richness covaries with forest age. Global Change Biology 25: 2446–2458.
United Nations Framework Convention on Climate Change. 2015. COP 21 Climate Agreement (UNFCCC, Paris), available at unfccc.int/resource/docs/2015/cop21/eng/l09r01.pdf.
Zhou, G. et al. 2006. Old-growth forests can accumulate carbon in soils. Science 314(1): 1417.
Read Next
December 2021
In this issue of IJW, we remember George Stankey and his contributions to wilderness research and stewardship. Mark Anderson provides a synthesis of recent findings on carbon storage in old growth forests. Rosemary Evans examines prescribed burning in Britain’s moorlands. And Tobias Nickel presents a call for a standard definition of “Natural” in wilderness stewardship.
Crises of Use
As we transition to a post-pandemic society, demand for transformational, restorative, and education experiences in nature will not recede. Nature has demonstrated its diverse benefits to a greater constituency these past months, and we as advocates, scientists, and managers need to embrace the challenge that comes with a larger audience.
George Stankey: Mentor, Colleague, Friend
George Stankey was a critical thinker who saw the big picture and could work across disciplinary boundaries.