Education & Communication
December 2017 | Volume 23, Number 2
by JOHN D. ROTHLISBERGER, TAMARA HEARTSILL SCALLEY, and RUSSELL F. THUROW
PEER REVIEWED
Editor’s Note: The U.S. Forest Service awarded the authors of this article with the 2017 National Award for Excellence in Wilderness Stewardship Research, proudly recognizing them for the research reported here and their outstanding contribution to wilderness and wild and scenic rivers management.
Abstract: Formerly diverse and abundant freshwater species are highly imperiled, with higher extinction rates than many other taxonomic groups worldwide. In the 50 years since passage of the US Wild and Scenic Rivers Act, wild and scenic rivers (WSRs) have contributed significantly to the conservation of native aquatic biodiversity as well as to the conservation and restoration of essential habitats. WSRs also have limitations. Conditions that impair aquatic species are not fully mitigated by WSR designation, although there are ways to reduce these limitations.
The US Wild and Scenic Rivers Act (WSRA) seeks to preserve “selected rivers or sections thereof in their free-flowing condition to protect the water quality of such rivers and to fulfill other vital national conservation purposes.” The mention of fulfilling “other vital national conservation purposes” hints at the essential role free-flowing rivers play in the conservation of native aquatic species. In the 50 years since passage, multiple lines of research have documented the importance of unobstructed rivers and natural hydrologic and disturbance regimes for the maintenance of aquatic biodiversity (Pringle 2000).
Wild and scenic rivers (WSRs) must be free-flowing and possess one or more outstandingly remarkable values (ORVs). Once designated, WSRs become part of the National Wild and Scenic Rivers System which protects their free-flowing condition, and maintains or enhances their water quality and ORVs. Outstandingly remark- able values encompass “scenic, recreational, geologic, fish and wildlife, historic, cultural, or other similar values.” A river’s ORVs must be unique, rare, or exemplary relative to its geographic context. Each WSR has a formal Comprehensive River Management Plan (CRMP) that identifies management objectives and practices designed to maintain and enhance its ORVs (Diedrich 2002; McGrath 2014). Section 10 of the WSRA emphasizes that WSRs are intended to protect “esthetic, scenic, historic, archaeologic, and scientific features,” all of which must be addressed in the CRMP.
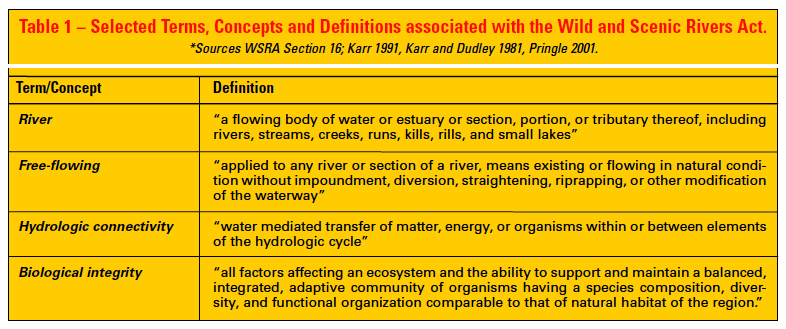
The three types of WSR classifications (Wild, Scenic, or Recreational) depend on the degree of anthropogenic development along a river. River management objectives depend on WSR type, but in all cases CRMPs seek to conserve and enhance river qualities and conditions for present and future generations. The WSR Wild classification includes those rivers or sections of rivers that are free of impoundments and generally inaccessible except by trail, with watersheds or shorelines essentially primitive and waters unpolluted. Those classified as Scenic must be free of impoundments, with shorelines or watersheds still largely primitive and shorelines largely undeveloped, but accessible in places by roads. Lastly, those classified as Recreational are readily accessible by road or railroad, have some development along their shorelines, and may have undergone some impoundment or water diversion in the past. Thus, CRMPs include both monitoring and management activities, as well as restrictions on incompatible uses, and may also include provisions for restoration activities (Haubert 1998). For example, if existing structures do not impede a river’s free flow (see Table 1), the presence of low dams, diversion works, and other minor structures prior to designation do not preclude a river from being designated as a WSR (Diedrich 2002). The restoration objective of removing such structures, therefore, may appear in a river’s CRMP.
Although the Forest Service (USFS), National Park Service (NPS), Bureau of Land Management (BLM), and Fish and Wildlife Service (USFWS) are the primary agencies managing WSRs, administration occurs by integrating authorities and coordinating actions of states, tribes, and 10 additional federal agencies with jurisdiction over various aspects of the management of water, river substrates, and adjacent riparian areas (Diedrich 1999). Currently, the USFS is the lead management agency for more river kilometers in the national system than any other agency (7,981 km [4,959 miles]) (Table 2). Protecting riparian areas and their immediate surroundings is essential to protecting the biologically favorable values and conditions of rivers. Stewardship of WSRs is consistent with the USFS mission of “securing favorable conditions of water flows,” which is embodied in the National Forests Organic Act of 1897. WSR designation encompasses rivers and adjacent lands, providing a riparian protection and management corridor that is on average 0.4 km (0.25 miles) wide. Consequently, the WSRA is proactive in recognizing the fundamental connection between aquatic and terrestrial systems.
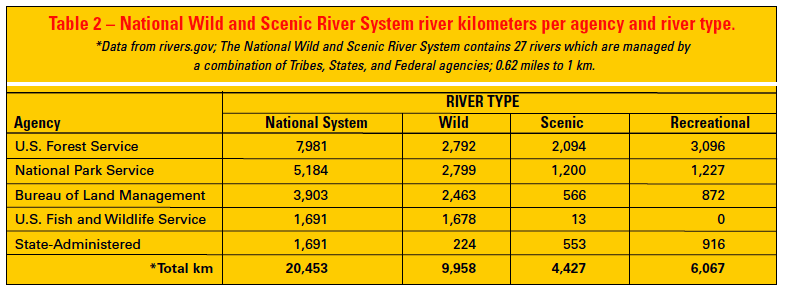
A river’s assemblage of fishes may qualify as an ORV, especially when threatened or endangered species, or Endangered Species Act (ESA)–designated critical habitat for such species, are present. Remarkable native species diversity or habitat diversity may also qualify as ORVs. Thus, conserving aquatic biodiversity may be among the primary purposes for WSR designation and can feature prominently in CRMPs. All WSRs, regardless of their identified ORVs, provide important benefits to aquatic communities. Even when conserving biodiversity is not specifically a goal of WSR designation, protecting rivers is the freshwater equivalent of creating preserves to protect terrestrial biodiversity. In this article, we briefly describe the aquatic resources of the United States, the threats they face, their conservation needs, and how WSRs contribute to meeting those needs. We also share examples of how specific WSRs benefit native species. Finally, we discuss limitations of WSRs and opportunities to improve their contributions to the conservation of biodiversity.
Aquatic Biodiversity and Threats
North American (NA) rivers and streams support a wide variety of aquatic and semi-aquatic flora and fauna. These organisms are taxonomically diverse and include microbes, algae, vascular plants, insects, mollusks, crustaceans, amphibians, reptiles, birds, mammals, and fishes. NA ecosystems are notable for their richness and diversity of species, and NA ranks first among continents for freshwater biodiversity (Master et al. 1998). Furthermore, NA aquatic biodiversity is globally critical for the conservation of various taxa. For example, 60% of all world crayfish species are native to NA (Crandall and Buhay 2008), and NA supports approximately 300 species of freshwater mussels, which account for 35% of 856 described global species (Graf and Cummings 2007). Approximately 10% of known freshwater and anadromous fishes are native to NA (801 species) (Master et al. 1998).
Many aquatic species in NA are endemic (i.e., found nowhere else) to a watershed or set of watersheds. Diverse NA ecosystems, ranging from coastal cypress swamps to glacial lakes to desert rivers to high-elevation streams, create unique habitats that contribute to high endemism rates. Large and small differences in the environmental conditions to which species evolutionarily adapted, have been maintained for geologically long periods of time resulting in a diverse set of unique aquatic species, each on its own evolutionary trajectory. Consequently, conserving populations of aquatic species in one region of the continent does not adequately provide for the maintenance of overall aquatic biodiversity.
Freshwater species are consistently recognized as among the world’s most imperiled taxa (Strayer and Dudgeon 2010). Freshwater fishes have higher extinction rates than other vertebrate taxa world- wide, and the current extinction rate for freshwater fishes in NA is nearly 900 times greater than the historical background rate (Burkhead 2012). In the United States, more than 75 fish species and numerous other aquatic species are designated as threatened or endangered. Endemic species, those confined to a small geographic range, or those with small populations are particularly at risk. To prioritize aquatic conservation efforts, it is essential to identify critical habitats and life his- tory requirements of at-risk species.
Multiple threats and stressors have caused historical declines in species diversity and abundance. North American waterways teemed with aquatic life prior to European settlement. For example, prior to the mid-19th century, huge Atlantic salmon runs entered northeastern US rivers (Webster 1982). Native salmon are no longer abundant in the eastern United States and some populations are endangered (Parrish et al. 1998). The Columbia River Basin was formerly the “most productive Chinook salmon habitat in the world” (Van Hyning 1973), with up to 16 million salmon returning annually. Current wild salmon returns represent about 2% of historical runs (Williams 2006). In undegraded Alaskan rivers, large runs of Chinook, sockeye, pink, chum, and coho salmon persist. Other species, such as freshwater mussels, were also abundant until the early 20th century (Haag 2012). Clench (1925, cited in Haag 2012) visited the Green River in Kentucky and reported “a bed of live shells […] over 300 feet long and 50 feet wide; they were so thick I could have filled a freight car.” Some previously abundant North American mussels are now threatened or endangered, others are extinct, and few species are abundant (Williams et al. 1993).
A variety of threats continue to affect aquatic and semi-aquatic species. These include habitat frag- mentation that limits gene flow and access to critical habitat (Nilsson et al. 2005); water withdrawals and other hydrologic alterations that change the timing and abundance of water, interfering with species’ ability to grow, migrate, and reproduce (Pringle 2001; Bunn and Arthington 2002); modifications of channels linked to agricultural, industrial, suburban, and urban land uses; changes in water temperature result- ing from thermal pollution, decreases or alterations of riparian cover, and climate change (Rieman and Isaak 2010); chemical pollution (Dudgeon et al. 2006; Rockström and Karlberg 2010); nonnative invasive species that modify native species assemblages through introgression, predation, competition, and habitat modification (Strayer 2010); and increased susceptibility to pathogens and diseases (Snieszko 1974).
With such a daunting array of threats, it is rarely effective to address problems individually. Moreover, given the interconnectedness of ecosystems and the hierarchical nature of aquatic systems embedded within terrestrial landscapes, it is rarely possible to solve one problem in isolation of others. Therefore, conservation efforts that affect large geographic areas and address entire ecosystems are much more likely to benefit species than more narrowly focused efforts (Williams et al. 2011). Although there is no minimum segment length for WSR designation and it is possible for individual WSR designations to be too small to protect biodiversity, when implemented at ecologically significant scales, designation and management of WSRs is consistent with an effective, broadscale, holistic approach (Pahl-Wostl et al. 2008; Cid and Pouyat 2013).
Aquatic Conservation Needs
Considering the plethora of aquatic taxa found in North American rivers, here we illustrate the conservation needs of one group by focusing on freshwater fishes, including potamodromous, anadromous, catadromous, and amphidromous forms (Morais and Daverat 2016). Schlosser (1991) provides a synthesis of freshwater fish life stages: life begins when fertilized eggs are either buried within substrates, broadcast over the surface of substrates, broadcast into the water column, or attached to plant material. Eggs mature after an incubation period lasting from a few days to several months when eggs hatch into embryos. When the embryo phase is complete, fish begin feeding on external energy sources and become larvae. When fully formed, larvae become juveniles that undergo many seasonally favorable periods of rapid growth, followed by seasonally less favorable growth periods. In temperate streams, favorable and unfavorable periods frequently involve migration between summer and winter habitats. Juveniles ultimately develop into subadults, the life stage immediately prior to sexual maturity. After sexual maturity, adults complete spawning migrations, locate appropriate sites for egg deposition, and reinitiate the life cycle. Within these general life stages, tremendous diversity occurs in life history characteristics of various species. For example, substantial variation occurs in spawning migrations; seasonal occurrence of eggs, young, and adults; and feeding habitats. Consequently, no single life history definition is all-inclusive.
The interrelationships that have evolved between these diverse species, life stages, and life history strategies and their habitats is complex. Scott and Crossman (1973) and Meehan (1991) provide detailed descriptions of the life stages and habitat requirements of many North American fishes and salmonids, respectively. The authors illustrate unique temporal and spatial habitat requirements of various life stages and life history types. In general, to persist, fishes require the maintenance of natural processes (e.g. fires, floods, debris flows) that operate on landscapes, deliver wood and sediment, alter stream channels, and create complex habitats (Reeves et al. 1995). Specifically, species require habitat components, including connectivity that enables life stages to move among suitable habitats; sufficient quantities of water to sustain living space and food sources; water of suitable chemical quality; a natural hydrological regime to maintain the conditions species and life stages evolved with; suitable water temperatures, including daily and seasonal temperature fluctuations; complex habitats exhibiting diverse substrates for life stages ranging from incubating embryos to overwintering adults; instream cover components such as large woody debris, aquatic plants, and algae; and appropriate native riparian and upland terrestrial vegetation. Each of these components is critical for maintaining the food webs and essential habitats necessary for species with a variety of life stages and diverse life history strategies to persist.
Contributions of Wild and Scenic Rivers to Aquatic Conservation
By their free-flowing character and the maintenance and enhancement of their ORVs, WSRs provide relatively intact and complex aquatic and riparian habitat and unimpaired water quality necessary for the long-term persistence of aquatic species. To illustrate the benefits of WSRs for aquatic biodiversity conservation, we provide examples from a temperate (central Idaho) and a tropical (Puerto Rico) region, and briefly describe contributions of WSRs for advancing knowledge, and their potential connections to educational and outreach activities.
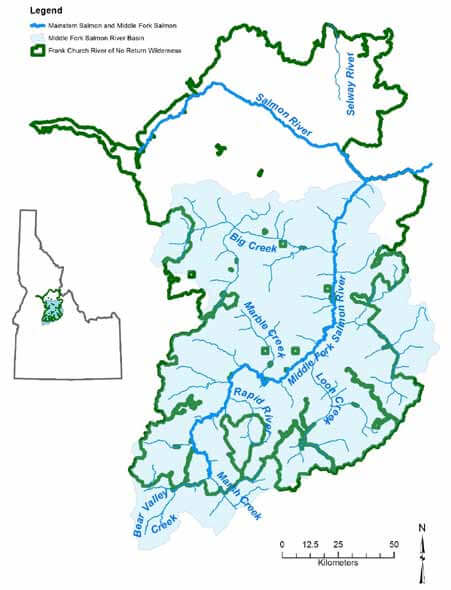
Insights from Central Idaho
Central Idaho’s Middle Fork Salmon River (MFSR) flows through the heart of the Frank Church–River of No Return Wilderness (FC– RNRW) (Figure 1). In 1968 the MFSR was one of the original eight rivers designated during creation of the National Wild and Scenic Rivers System. From its origin, the MFSR flows north-northwest for 171 kilometers (106 miles) through the Salmon River Mountains and joins the Salmon River. Twelve major streams and hundreds of smaller ones are tributary to the river. From 1930 to 1980, a majority of the region was managed in “primitive area” status (USFS Service 1998). In 1980, the Central Idaho Wilderness Act established the 906,136-hectare (2.2 million acre) wilderness that remains the largest contiguous wilderness in the lower 48 states and the largest in the national forest system. The Central Idaho Wilderness Act also added the main stem Salmon River to the National Wild and Scenic River System.
Landscapes are dynamic systems shaped by a variety of natural processes across a range of spatial and temporal scales. In central Idaho, processes include spring snowmelt and peak flows, winter rain-on-snow events and floods, snow avalanches, windstorms, droughts, wildfires, high intensity rainstorms, landslides and debris flows, earthquakes, and extreme temperatures. Natural processes play a critical role in creating aquatic habitat complexity, diversity, and connectivity (Reeves et al. 1995; Dunham et al. 2002). Although human activities have degraded most landscapes and altered natural disturbance regimes, the FC–RNRW is an exception. Its protected status enables natural processes to function relatively unimpeded by anthropogenic activities across its extensive landscape (Figure 2). Natural processes create and maintain a dynamic mosaic of landscape conditions that support diverse, high quality, and connected habitats (Thurow 2015). Because of its large size, functioning natural processes, and the diverse, high quality, connected habitats they create, a nearly complete native species assemblage persists in the Frank; only grizzly bears and Indigenous people are absent from the region Lewis and Clark explored in 1804 (Thurow 2015).
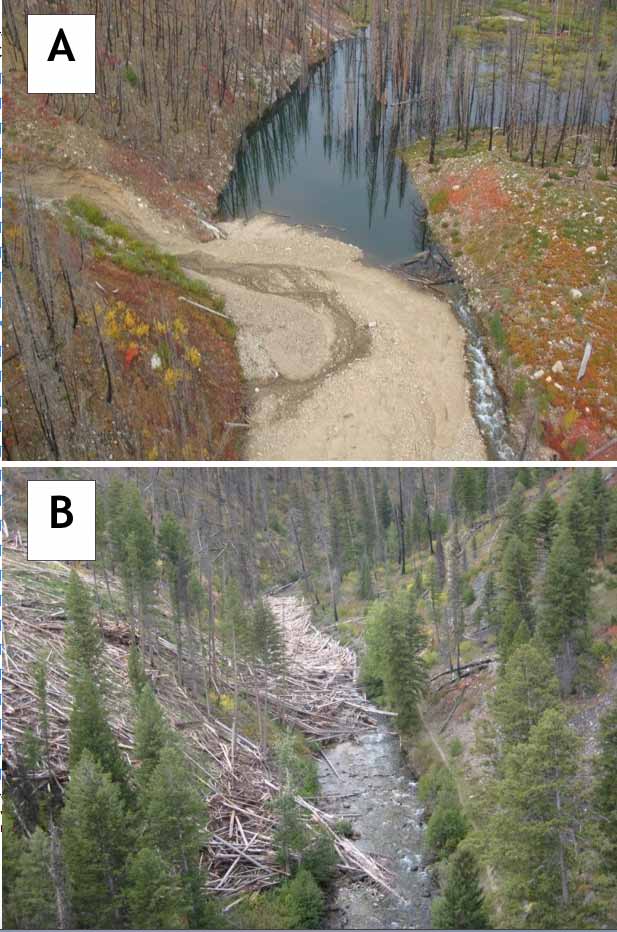
To illustrate these processes, since the mid-1980s, climate-driven increases in the size and severity of wildfires in the western United States (e.g., Westerling et al. 2006) have profoundly affected forested and aquatic ecosystems. High fuel densities, combined with drought, caused forests to be highly susceptible to changes in the timing of snowmelt and increased the length of the fire season (Westerling et al. 2006). Since 1990, wildfires have burned extensive portions (>52%) of the MFSR Basin (Thurow 2015). Paleo records of fire confirm that intense fires have been a natural part of this landscape for thousands of years (Pierce et al. 2004; Svenson 2010; Whitlock et al. 2010). Fire-related debris flows have been an integral part of the FC–RNRW for at least 12,000 years (Riley et al. 2011). Intense thunderstorms or rain-on- snow events postfire create debris flows or snow avalanches that recruit wood, carbon, and sediment to streams. Runoff-generated, fire-related debris flows (Meyer and Wells 1987) from tributaries contribute immense volumes of sediment (Kirchner et al. 2001; Riley et al. 2011). Such events also occur in unburned, geologically unstable landscapes. Inputs of fine- particulate carbon and large woody debris (LWD) are critical for aquatic ecosystems; LWD provides essential channel structure, habitat diversity, nutrient retention, and carbon to streams (Bilby and Likens 1980; Bilby 1981; Seo et al. 2008; Martin and Benda 2001), and fine organic carbon forms the basis of aquatic food webs for primary and secondary consumers (Mulholland and Watts 1982).
Pacific salmon and other aquatic species have evolved in these dynamic freshwater environments. A complex suite of disturbance patterns and processes create and alter essential habitats to provide a physical template essential to the expression of native species life history and genetic diversity. Chinook salmon, for example, persist through a host of adaptations such as multiple life history strategies, high fecundity, extended incubation, high mobility, and straying to new spawn- ing sites (Figure 3) (NRC 1996). Survival in dynamic environments is also a matter of scale; persistence will be influenced by how well dispersal dynamics and reproductive rates merge with scales of disturbance on the landscape. The exceptional resilience, flexibility, and diversity that characterize salmon are fundamental to their wide distribution, historic abundance, and value (Martin and Glick 2008). Life history diversity increases production and buffers population fluctuations (Greene et al. 2010; Healey 2009). Within the FC– RNRW, multiple fresh- and saltwater Chinook salmon ages may provide up to 18 different age classes spawn- ing each year (Gebhards 1960; James et al. 1998; Copeland and Venditti 2009). Despite extremely low populations levels (Thurow 2000), salmon populations retain both within- and across-tributary population differentiation (Neville et al. 2006a).
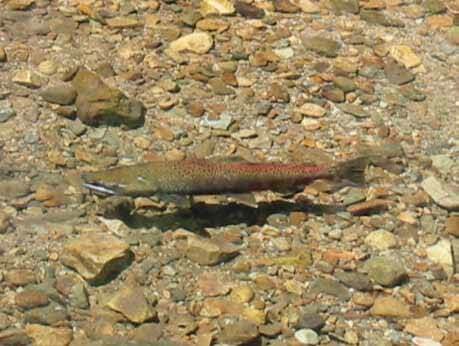
Wild, indigenous Chinook salmon and summer steelhead populations such as those in the FC–RNRW are rare; Thurow et al. (2000) reported their presence in 4% and 10% of the potential historical range, respectively, and 15% and 22% of the current range, respectively, in the Columbia River Basin and portions of the Klamath River Basin. Most other wild populations were either extirpated by impassable dams or have been supplemented with hatchery-reared fish (Thurow et al. 2000). Federal fisheries management agencies identified “four H’s” (habitat degradation, harvest, hatchery practices, and hydrosystem operation) as the primary causes of anadromous fish declines (NMFS 2000). Construction and operation of main-stem dams on the Columbia and Snake Rivers, however, is considered the proximate cause of anadromous fish declines (CBFWA 1990). The adverse effects of main- stem dams and impoundments on salmon and steelhead survival were well documented (Raymond 1979), and by the early 1990s, all wild Snake River Chinook salmon and steelhead populations were federally listed under ESA. Despite abundant, high quality natal habitat; absence of hatchery fish; and low ocean harvest rates verified by tag returns, MFSR Chinook salmon and summer steelhead remain at risk of extirpation, primarily because of outside basin factors in the Columbia River and Snake River migration corridor, estuary, and ocean. Today, all anadromous fish in the Salmon River basin must navigate eight dams (four in the Columbia River and four in the lower Snake River) to reach the ocean as smolts and ascend the dams as adults returning to spawn. Because of MFSR population declines, angling has been closed for Chinook salmon and steelhead since 1978. In the 2016 Draft Chinook Salmon and Steelhead Recovery Plan, NOAA reported that “[f ]ederal hydropower projects in the lower Columbia and Snake Rivers remain a primary threat to the viability of Snake River spring/summer Chinook salmon and steelhead.”
Native salmonids have generally persisted in the areas least influenced by humans (Thurow et al. 1997). Within the western United States, the strongest and most intact native fish populations occur within a network of federally protected and managed lands, including WSRs, roadless and wilderness areas, and national parks (Williams et al. 2011). Protected areas anchor population strongholds (Lee et al. 1997) and function as refugia. The Frank contains high quality, diverse, and functioning aquatic habitats that provide strongholds for 15 native fishes. A host of other federally listed species, recently federally delisted species (peregrine falcon, bald eagle, and gray wolf), and US Forest Service Regions 1, 4-Sensitive Species and sensitive native plants depend on the Frank and its natural processes for essential, core habitats (Thurow 2015).
Greene et al. (2010) and Haak and Williams (2012) emphasized the importance of such biocomplexity and suggested maintaining diverse life history portfolios of populations may be crucial for their resilience to unfavorable conditions such as cli- mate change. Hilborn et al. (2003) described how the preservation of bio- diversity in Alaskan sockeye salmon population has been attributed to the species’ ability to persist and support fisheries in a changing climate. Rieman and Isaak (2010) summarized potential responses to climate change and emphasized maintaining biodiversity to ensure the greatest capacity for natural biological adaptation to variable and changing environments. Crucial steps for conserving biodiversity include (1) maintain- ing connectivity to allow gene flow, retain genetic diversity, and allow recolonization (Dunham and Rieman 1999; Isaak et al. 2007; Neville et al. 2006b); (2) conserving the fullest range of genetic and phenotypic diversity (Rieman and Isaak 2010); and (3) maintaining spatial structure and redundancy to retain phenotypic and genetic diversity (Allendorf et al. 1997; Healey 2009).
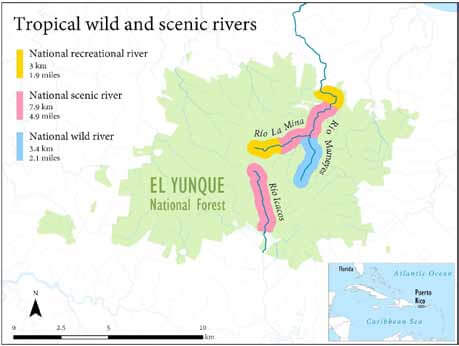
Large, well-connected, high-elevation, aquatic habitats may also serve as anchors for species survival and recovery in the era of global warming (e.g., Martin and Glick 2008). Isaak et al. (2016) reported that the relatively slow pace of water temperature increases in high elevation mountain streams portends their role as refugia for cold-water biodiversity. The MFSR’s importance as a cold- water refugia is further enhanced by its unique salmon that spawn at the highest elevations of any spring/sum- mer Chinook salmon population in the world (Crozier et al. 2008).
Insights from Puerto Rico
The National System includes three tropical rivers, all located within the El Yunque National Forest of Puerto Rico (USFS 1993). The Río Mameyes, the Río La Mina, and the Río Icacos were each designated in 2002 (Figure 4). These WSR designations maintain the free-flowing condition of these rivers, which are among the last remaining unobstructed rivers in Puerto Rico (Figure 5). The WSR designations also secure flows that allow migratory native aquatic fauna to complete their life cycles. Although a majority of the designated WSR areas occur within the national forest, significant portions are located outside of forest boundaries (Heartsill Scalley and López-Marrero 2014). These three rivers dissect terrain with steep hillslopes where flash floods, overland flows, and landslides occur regularly. Such natural disturbances maintain and create high quality habitat for native fauna (Lugo and Heartsill Scalley 2014; Ortíz-Zayas and Scatena 2004).
Aquatic fauna in these WSRs are amphidromous, residing in freshwater habitats as adults and in estuarine or coastal/marine waters during early life stages (Pringle 2000). Native fishes, shrimp, and snails migrate among headwater streams, rivers, and coastal estuaries to reach the Caribbean Sea or Atlantic Ocean (Cook et al. 2009; Covich et al. 2006; Covich and McDowell 1996) (Figure 6). After rearing in salt water, they migrate back upstream as postlarvae to occupy pools in streams along elevational gradients in the WSRs (Heartsill Scalley et al. 2001; Greathouse et al. 2006; Harris et al. 2012). Ten native species of shrimp, at least six fishes, various snails, and one endemic fresh- water crab (buruquena) inhabit these WSRs. The diverse shrimp species dominate the aquatic fauna biomass in these tropical WSRs and streams (Harris et al. 2012). Four shrimp species are filter-feeders and scraper- grazers before (gata and guabara) while another species, the salpiche, is a shredder and particle feeder (Crowl et al. 2006). The palaemonid genus Macrobrachium includes five species, such as bocú and zurdo, which are the predators and omnivores feed- ing on leaf detritus, fine particulate organic matter, macrophytes, algae, small invertebrates, mollusks, small fishes, and other shrimp (Covich and McDowell 1996). Fishes are also predominantly amphidromous, although recent findings reveal there is variation in the migratory strategies of these native fish assemblages (Kwak et al. 2013). Fishes include omnivorous gobies, saga, and various species of algivorous gobies, sirajo or chupapiedra; the eleotrids, big-mouth sleeper, also known as guavina, and the spiny-cheek sleeper; a freshwater dajao mullet; and American eel. Native diadromous freshwater snails such as the burgao are also found in the WSRs. These snails are so sensitive to water depth that they are not found in rivers that are hydrologically disconnected from the ocean or that exhibit excessive accumulation of estuary sediments (Blanco and Scatena 2006). The WSRs in the El Yunque National Forest maintain fundamental ecosystem components including continuous stream dis- charge, riparian vegetation, naturally heterogeneous channel substrates, and hydrologic connectivity between headwaters and designated river sections. The Puerto Rico Department of Natural Resources has developed river protection designations that would extend and complement the free- flowing condition of the WSR section of the Río Mameyes to ensure river connectivity beyond federal lands, all the way to its estuarine sections and its coastal outlet.
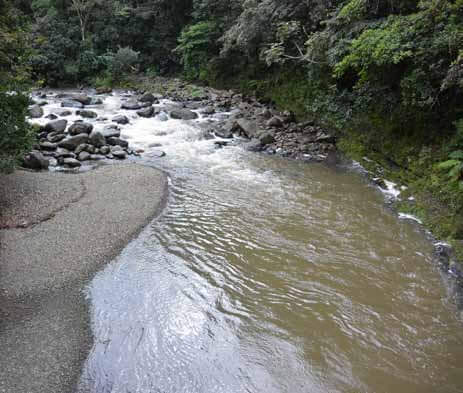
Despite differences between tropical Puerto Rican and intermountain western rivers and their fauna, the designation and management of WSRs in both regions is essential for native species persistence. WSRs ensure that high quality, connected, and naturally functioning aquatic habitats are retained for native species. In both locales, WSRs act as refugia for at-risk aquatic species and help conserve significant elements of aquatic biodiversity.
Advancement of Knowledge
Altered landscapes compromise our ability to examine natural processes and species responses. Wilderness offers unique opportunities for social and biophysical research in locations relatively unmodified by humans. Within the confines of watersheds that hierarchically incorporate both wilderness and nonwilderness areas, WSRs provide the minimum accumulation of anthropogenic effects at the landscape scale. However, because rivers from their headwaters to their drainage outlets are integrated into multiple-use landscapes, true wilderness in WSRs depends on the spatial context and scale of the section of the river being designated. Nevertheless, both wilderness areas and WSRs provide locations for passively examining natural processes and species in the absence of confounding management and land use. Consequently, such areas function as controls, baselines against which we may measure the
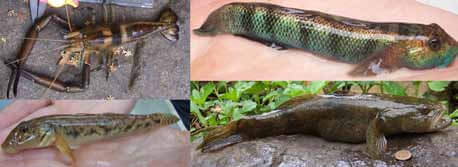
Altered landscapes compromise our ability to examine natural processes and species responses. Wilderness offers unique opportunities for social and biophysical research in locations relatively unmodified by humans. Within the confines of watersheds that hierarchically incorporate both wilderness and nonwilderness areas, WSRs provide the minimum accumulation of anthropogenic effects at the landscape scale. However, because rivers from their headwaters to their drainage outlets are integrated into multiple-use landscapes, true wilderness in WSRs depends on the spatial context and scale of the section of the river being designated. Nevertheless, both wilderness areas and WSRs provide locations for passively examining natural processes and species in the absence of confounding management and land use. Consequently, such areas function as controls, baselines against which we may measure the results of management experiments (Noss 1991).
The FC–RNRW provides a natural laboratory for USFS scientists and collaborators to examine a host of research topics: the role of debris flows and avalanches in contributing carbon and wood to streams (Figure 2); validating methodologies and sampling designs for salmon population monitoring; examining linkages between fine-scale genetic structure, demographic parameters, and environmental characteristics; assessing salmon dispersal and environmental constraints using spatial autocorrelation; validating hydrologic models that predict spawning gravel distribution; monitoring salmon responses to stochastic events; assessing environ- mental covariates affecting salmon habitat occupancy; and evaluating changes in salmon phenology in response to a changing climate. Similarly, the Mameyes WSR (Figure 5) provides a unique opportunity to monitor shrimp population dynamics, organic matter export, and water quality in a free-flowing system (Scatena and Johnson 2001; Pérez-Reyes et al. 2015; Heartsill Scalley et al. 2012). Long-term datasets collected in Puerto Rican WSRs have been instrumental in improving our understanding of the effects of disturbance caused by flow alterations and the faunal extirpations and ecosystem process disruptions that occur in dammed and modified rivers (Greathouse et al. 2006; Crook et al. 2009). Research values of WSRs may be enhanced by completing and curating accurate stream length data among WSR management agencies. Current deficiencies in spatial data include WSR boundary delimitations and stream length data at appropriate regional and local scales (McManamay 2013). The Interagency WSR Coordinating Council has also identified the need for a comprehensive database of aquatic species occurrence, legal status, their habitat, and other management-significant ecological data.
WSRs may also assist efforts to connect people with and edu- cate them about landscapes. Many people who visit WSRs identify them as providing a unique sense of place that is inherently valuable for their well-being (Smith and Moore 2011). Communities local to WSRs also perceive recreational, resource, social, psychological, and economic benefits (Smith and Moore 2011). Much untapped potential remains to expand the role of WSRs in educational and outreach activities connected to ongoing research efforts in these watersheds.
Limitations of Wild and Scenic Rivers for Aquatic Conservation
Despite its considerable value, the WSRA by itself is not a comprehensive tool for conserving aquatic biodiversity. Although the act contributes to maintaining hydrological connectivity of designated rivers, only 0.3% of America’s river kilometers are designated as Wild and Scenic (Benke 1990). The WSRA also does not prohibit development (e.g., hydropower projects, water withdrawal) below or above WSR segments. Therefore, a key limitation of WSRs is their inability to protect all life stages of all aquatic species contained in entire watersheds. Consider wide-ranging species such as anadromous fish, which spawn in freshwater, migrate to the ocean, and rear in the marine environment. Although WSRs may protect spawning and natal habitat, essential migratory corridors and estuaries that fall outside designated river segments may be managed separately. For example, despite the MFSR’s excellent natal habitat and uniquely adapted fish, because of factors outside the WSR, all anadromous salmonids in the drainage are ESA listed and at risk of extirpation (Thurow 2000). Disturbances from dams and water withdrawals in lower portions of watersheds are also transmitted to upstream systems by reducing genetic flow among source and sink populations and altering nutrient cycling and primary productivity (Greathouse et al. 2006; Pringle 1997). In the tropical Río Mameyes, it is necessary to find partners outside the WSR to promote actions that limit riparian area land use and land cover change. Riparian areas in this WSR have the second largest percentage of urbanization of all the rivers originating in El Yunque National Forest (Heartsill Scalley and López-Marrero 2014). Land cover changes associated with recreation access, pollution and contamination from recreational activities, and hydrological changes in the lowland and coastal areas are stressors to this river system.
For the full conservation benefits of WSRs to be realized, management must be coordinated across jurisdictions using multiple authorities. In most cases, only a portion of a river receives WSR designation, so upstream and downstream conditions and management objectives in undesignated sections influence aquatic biodiversity in the protected segments (McManamay et al. 2012). In recent years, there has been increasing recognition of the importance of managing rivers at the watershed scale. For example, in 2009, Congress designated partial watershed-scale WSRs for the upper Snake River (WY), the Owyhee River (ID), and the Virgin River (UT). The location of the protected WSR segment within a watershed (i.e., lower, middle, upper) will bring with it management issues and challenges. Designated segments in lower water- sheds portions may become sediment deficient if sediment is retained by upstream dams. Water extraction in upstream river segments may also affect downstream segments (Pringle 2001). WSR segments in middle sections of a watershed may experience degradation from upstream and downstream segments. Groundwater pumping, changes in terrestrial vegetation, and elimination of natural flooding regimes commonly alter middle watershed areas. Designated WSRs in upper watersheds are usually in mountainous or wilderness areas. These upper watershed WSRs are vulnerable to the effect of isolation and diminished hydrological connection to their lower watersheds.
An important shortcoming of the WSRA with respect to the water law of the western United States and the conservation of aquatic biodiversity is that designated river segments can be de-watered if senior water rights are present higher in the water- shed. The WSRA protects instream flows only to the extent made possible by the reservation of a federal water right with a priority date that is the same as the date of the river segment’s designation. This makes the reserved federal water rights of most WSRs very junior ones and therefore does little to protect or augment instream flows. This shortcoming can be partially overcome, however, by seeking to ensure wherever possible that WSR designations encompass the headwaters of a river’s main stem and those of its critical tributaries.
Nonnative species may not be considered in the designation of WSRs, and unless an aquatic or terrestrial species wildlife ORV is recognized as potentially impaired by nonnative species, a river’s CRMP is unlikely to address the prevention and management of nonnative species. State agencies also typically retain authority to manage fish and wildlife populations in both WSRs and designated wilderness. The Central Idaho Wilderness Act of 1980, for example, states: “[N]othing in this Act shall be construed as affecting the jurisdiction or responsibilities of the State of Idaho with respect to wildlife and fish in the national forests in Idaho.” Despite the state’s authority, stocking of alpine lakes in tributaries to the MFSR WSR is guided by a Memorandum of Understanding between the Idaho Department of Fish and Game (IDFG) and Forest Service. Historically, many of the lakes in the FC–RNRW were fishless and began receiving fish in the early 1900s when stocking was conducted by backpack and horseback; this has been followed by aerial stocking in the last 50 years (IDFG 2013). IDFG (2013) notes that historical alpine lake management was conducted to provide diverse angling opportunities, and little consideration was given to native lake fauna prior to wilderness designation. Fish introductions in some barren lakes have reduced native amphibian populations through predation and competition (Hoffman and Pilliod 1999). As a result, in recent years, IDFG has developed an adaptive management approach to guide the Frank’s alpine lake fish-stocking pro- gram. Information from a variety of agencies and sources is incorporated, and ecological and biological aspects of maintaining native amphibian and downstream fish populations are considered in determining how alpine lakes are managed (IDFG 2013).
In areas where management objectives and priorities are not aligned with conservation goals, lack of consideration for nonnative species may limit the contributions of WSRs to the conservation of native aquatic biodiversity because nonnative species may affect native food webs and alter ecosystem functions or displace native species populations. Whether fish and wildlife are an ORV of a particular WSR, the value of WSRs for conserving native aquatic biodiversity would be improved if each CRMP addressed collaborative management of nonnative species. As in the example above, CRMPs could include provisions to avoid the stocking of nonnative fish into designated lakes and river segments. Providing for nonnative species management in WSRs is particularly important because people attracted to WSRs for recreation may inadvertently introduce nonnative species. Furthermore, the free-flowing condition of WSRs allow nonnative aquatic species that establish populations to spread upstream or downstream more readily than in rivers where barriers are present. Clearly, protecting or restoring the free-flowing condition of rivers is highly beneficial to the native species that are evolutionarily adapted to the physical characteristics of free-flow- ing conditions (e.g., temperature, turbidity, hydrograph). On the other hand, the physical conditions associated with regulated rivers (e.g., low turbidity, flattened hydrograph, and cold temperatures) often favor nonnative plants and animals (e.g., tamarisk, nonnative cold-water salmonids) (Johnson et al. 2008). Altered conditions that promote the establishment of nonnative species to the detriment of native species frequently occur below dams where WSR-designated river segments often begin. Such locations make the inclusion of nonnative species management in CRMPs even more compelling. WSRs may be particularly good candidates for efforts to prevent and control harmful nonnative species because WSR segments may experience fewer effects from other stressors (e.g., pollution, habitat fragmentation) than other rivers. Thus, benefits from successful nonnative species management may be less likely to be obscured by other environmental problems in WSRs than elsewhere (Abell et al. 2007).
Despite their protected status, some WSRs retain legacy effects from historical activities that occurred prior to designation (e.g., dredge mining). In such instances, habitat restoration projects provide an opportunity to enhance the “wild character,” free- flowing conditions, and water quality of the river and benefit aquatic species. However, constraints imposed on the management of WSRs may complicate efforts to enhance habitat quality for aquatic species. Environ- mental review standards for all WSR projects, including habitat management and restoration, are more stringent than for undesignated rivers. The WSRA requires the evaluation of any projects on a designated river section to ensure there are no “direct and adverse” impacts to the river (see Section 7 review, https://www.rivers.gov/documents/section-7.pdf ). To avoid unnecessary complications, WSR management agencies should work to promote consistency in project review standards, within and across agencies. Publications of the Interagency WSR Coordinating Council website pro- vide resources to facilitate this process (https://www.rivers.gov/publications.php).
Summary
Formerly diverse and abundant, native aquatic species and their habitats are at risk from a variety of threats. Despite their limitations, WSRs provide complex aquatic and riparian habitats, favorable hydrological conditions, and natural processes necessary for the long-term persistence of many aquatic species. Achieving sustainable use of freshwater resources for the benefit of present and future generations will require continued pursuit of actions that conserve and restore species and their habitats. McGrath (2014) suggests that a comprehensive approach inclusive of all stakeholders will tend to maximize the WSRA’s effectiveness. A comprehensive approach may include research, monitoring, a combination of protected areas and multiple-use areas, restoration projects, outreach, and educational initiatives (Dudgeon et al. 2006; McGrath 2014). The Interagency Wild and Scenic Rivers Coordinating Council was formed in 1993 to assist the development of an integrated approach transcending political, land, and water management boundaries (https://www.rivers.gov/ council.php). As Franklin (1993) observed, reserves will never be large enough or sufficiently distributed to maintain all biological diversity. Protection of aquatic strongholds, such as WSRs, will also not be sufficient on its own (Thurow et al. 2000). To conserve biodiversity, a combination of reserves, aquatic restoration efforts, instream flow provisions, and adoption of more ecologically compatible land use policies on adjacent lands (e.g., Thurow et al. 1997) will be required (Abell et al. 2007).
Acknowledgments
We thank H. Wadsworth, E. Lugo, and two anonymous reviewers whose suggestions improved this manuscript. S. Boucher provided data on partnerships associated WSRs. P. Rios and J. Ortega shared docume tation about designation of WSRs in the El Yunque National Forest. All research at the International Institute of Tropical Forestry, USDA Forest Service, is done in collaboration with the University of Puerto Rico. The findings, conclusions, and views expressed in this manuscript are those of the authors and do not necessarily represent the views of the US Forest Service.
JOHN D. ROTHLISBERGER, PhD, is national program leader for Fish and Aquatic Ecology Research, USDA Forest Service, Washington Office Research & Development; email: jrothlisberger@ fs.fed.us.
TAMARA HEARTSILL SCALLEY, PhD, is research ecologist for the USDA Forest Service, International Institute of Tropical Forestry in Rio Piedras, Puerto Rico.
RUSSEL F. THUROW, MS, is research fish biologist for the USDA Forest Service, Rocky Mountain Research Station in Boise, ID.
References
Allendorf, F. W., D. W. Bayles, D. L. Bottom, K. P. Currens, C. A. Frissell, D. Hankin, J. A. Lichatowich, W. Nehlsen, P. C. Trotter, and T. H. Williams. 1997. Prioritizing Pacific salmon stocks for conservation. Conser- vation Biology 11: 140–152.
Abell, R., J. D. Allan, and B. Lehner. 2007. Unlocking the potential of protected areas for freshwaters. Biological Conservation 134: 48–63.
Benke, A. C. 1990. A perspective on America’s vanishing streams. Journal of the North American Benthological Society 9: 77–88.
Bilby, R. E. 1981. Role of organic debris dams in regulating the export of dissolved and particulate matter from a forested watershed. Ecology 62: 1234–1243.
Bilby, R. E., and G. E. Likens. 1980. Importance of organic debris dams in the structure and function of stream ecosystems. Ecology 61: 1107–1113.
Blanco, J. F., and F. N. Scatena. 2006. Hierarchical contribution of river ocean connectivity, water chemistry, hydraulics and substrate to the distribution of diadromous snails in Puerto Rican streams. Journal of the North American Benthological Society 25: 82–98.
Bunn, S. E., and A. H. Arthington. 2002. Basic principles and ecological consequences of altered flow regimes for aquatic biodiversity. Environmental Management 30: 492–507.
Burkhead, N. M. 2012. Extinction rates in North American freshwater fishes, 1900–2010. BioScience 62: 798–808.
CBFWA (Columbia Basin Fish and Wildlife Authority). 1990. Integrated system plan for salmon and steelhead production in the Columbia Basin. Portland, OR: Northwest Power Planning Council.
Cid, C. R., and R. V. Pouyat. 2013. Making ecology relevant to decision making: The human-centered, place-based approach. Frontiers in Ecology and the Environment 11: 447–448.
Clench, W. J. 1925. Collecting in Kentucky. Nautilus 39: 71–72.
Cook, B. D., S. Bernays, C. M. Pringle, and J. M. Hughes. 2009. Marine dispersal determines the genetic population structure of migratory stream fauna of Puerto Rico: Evidence for island-scale population recovery processes. Journal of the North American Benthological Society 28: 709–718.
Copeland, T., and D. A. Venditti. 2009. Contribution of three life history types to smolt production in a Chinook salmon (Oncorhynchus tshawytscha) population. Canadian Journal of Fisheries and Aquatic Sciences 66: 1658–1665.
Covich, A. P., T. A. Crowl, and T. Heartsill Scalley. 2006. Effects of drought and hurricane disturbances on headwater distributions of palaemonid river shrimp (Macrou- brachium spp.) in the Luquillo Mountains, Puerto Rico. Journal of the North American Benthological Society 25: 99–107.
Covich, A. P., and W. H. McDowell. 1996. The stream community. In The Food Web of a Tropical Rain Forest, ed. D. P. Reagan and R. B. Waide (pp. 433–459). Chicago: University of Chicago Press.
Crandall, K. A., and J. E. Buhay. 2008. Global diversity of crayfish (Astacidae, Cambaridae, and Parastacidae – Decapoda) in freshwater. Hydrobiologia 595: 295–301.
Crook, K. E., C. M. Pringle, and M. C. Freeman. 2009. A method to assess longitudinal riverine connectivity in tropical streams dominated by migratory biota. Aquatic Conservation: Marine and Freshwater Ecosystems 19: 714–723.
Crowl, T. A., V. K. Welsh, T. Heartsill Scalley, and A. P.Covich. 2006. The role of shrimp as shredders and litter source on leaf breakdown rates in tropical montane streams. Journal of the North American Benthological Society 25: 196–206.
Crozier L. G., A. P. Hendry, P. W. Lawson, T. P. Quinn, N. J. Mantua, J. Battin, R. G. Shaw, and R. B. Huey. 2008. Potential responses to climate change in organisms with complex life histories: Evolution and plasticity in Pacific salmon. Evolutionary Applications 1: 252–270.
Diedrich, J. 1999. Implementing the Wild & Scenic Rivers Act: Authorities and Roles of Key Federal Agencies. Technical Report of the Interagency Wild and Scenic Rivers Coordinating Council.
———. 2002. Wild & Scenic River Management Responsibilities. Technical Report of the Interagency Wild and Scenic Rivers Coordinating Council.
Dudgeon, D., A. H. Arthington, M. O. Gessner, Z.-I. Kawabata, D. J. Knowler, C. Lévêque, R. J. Naiman, A.–H. Prieur-Richard, D. Soto, M. L. J. Stiassny, and C. A. Sullivan. 2006. Freshwater biodiversity: Importance, threats, status and conser- vation challenges. Biological Reviews 81: 163–182.
Dunham, J. B., and B. E. Rieman. 1999. Metapopulation structure of bull trout: Influences of physical, biotic, and geometrical landscape characteristics. EcologicalApplications9: 642–655.
Dunham, J. B., B. E. Rieman, and J. T. Peterson. 2002. Patch-based models to predict species occurrence: Lessons from salmonid fishes in streams. In Predicting Species Occurrences: Issues of Scale and Accuracy, ed. J. M. Scott, P. Heglund, M. Morrison, J. Haufler, and B. Wall (pp. 327–334). Washington, DC: Island Press.
Franklin, J. F. 1993. Preserving biodiversity: Species, ecosystems, or landscapes? Ecological Applications 3: 202–205.
Gebhards, S. V. 1960. Biological notes on precocious male Chinook salmon parr in the Salmon River drainage, Idaho. The Progressive Fish-Culturist 22: 121–123.
Graf, D. L., and K. S. Cummings. 2007. Review of the systematics and global diversity of freshwater mussel species (Bivalvia: Unionoida). Journal of Molluscan Studies 73: 291–314.
Greathouse, E. A., C. M. Pringle, W. H. McDowell, and J. G. Holmquist. 2006. Indirect upstream effects of dams: Consequences of migratory consumer extirpation in Puerto Rico. Ecological Applications 16: 339–352.
Greene, C. M., J. E. Hall, K. R. Guilbault, and T. P. Quinn. 2010. Improved viability of populations with diverse life-history portfolios. Biological Letters 6: 382–386.
Haag, W. R. 2012. North American freshwater mussels: Natural history, ecology, and conservation. Cambridge, UK: Cambridge University Press.
Haak, A. L., and J. E. Williams. 2012. Spreading the risk: Native trout management in a warmer and less certain future. North American Journal of Fisheries Management 32: 387–401.
Haubert, J. 1998. An Introduction to Wild and Scenic Rivers. Technical Report of the Interagency Wild and Scenic Rivers Coordinating Council.
Harris, N. L., A. E. Lugo, S. Brown, and T. Heartsill Scalley, eds. 2012. Luquillo Experimental Forest: Research history and opportunities. Washington, DC: US Department of Agriculture, Forest Service, EFR-1.
Healey, M. 2009. Resilient salmon, resilient fisheries for British Columbia, Canada. Ecology and Society 14: 2.
Heartsill Scalley, T., T. A. Crowl, M. Townsend, and A. P. Covich. 2001. Freshwater shrimp population structure and distribution dynamics in a tropical rainforest stream. In Tropical Ecosystems: Structure, Diversity and Human Welfare (pp. 732–735). New Delhi: Oxford and IBH Publishing.
Heartsill Scalley, T., and T. del M. López- Marrero. 2014. Land-cover composition, water resources and land management in the watersheds of the Luquillo Mountains, northeastern Puerto Rico. Caribbean Geography 19: 43–68.
Heartsill Scalley T., F. N. Scatena, S. Moya, and A. E. Lugo. 2012. Long-term dynamics of organic matter and elements exported as coarse particulates from two Caribbean montane watersheds. Journal of Tropical Ecology 28(2): 127–139.
Hilborn, R., T. P. Quinn, D. E. Schindler, and D. E. Rogers. 2003. Biocomplexity and fisheries sustainability. Proceedings of the National Academy of Science 100: 6564–6568.
Hoffman, R. L., and D. S. Pilliod. 1999. The Ecological Effects of Fish Stocking on Amphibian Populations in High-Mountain Wilderness Lakes. Final Report. Corvallis, OR: USGS/BRD Forest and Rangeland Ecosystem Science Center.
Idaho Department of Fish and Game (IDFG). 2013. Fisheries management plan, 2013–2018. Boise, Idaho.
Isaak, D. J., R. F. Thurow, B. E. Rieman, and J. B. Dunham. 2007. Relative roles of habitat size, connectivity, and quality on Chinook salmon use of spawning patches. Ecological Applications 17: 352–364.
Isaak, D. J., M. Young, C. H. Luce, S. H. Hostetler, S. J. Wenger, E. E. Peterson, J. M. Ver Hoef, M. C. Groce, D. L. Horan, and D. E. Nagel. 2016. Slow climate velocities of mountain streams portend their role as refugia for cold-water biodiversity. Proceedings of the National Academy of Sciences 113:4374–4379.
James, B. B., T. N. Pearsons, and G. A. McMicheal. 1998. Washington Department of Fish and Wildlife, Spring Chinook Salmon Interactions Indices and Residual/ Precocial Monitoring in the Upper Yakima Basin. Annual Report to Bonneville Power Administration, Contract No. 1995B164878, Project No. 0906409, BPA Report DOE/ BP-64878-4.
Johnson, P. T., J. D. Olden, and M. J. Vander Zanden. 2008. Dam invaders: Impoundments facilitate biological invasions into freshwaters. Frontiers in Ecology and the Environment 6: 357–363.
Kirchner J. W., R. C. Finkel, C. S. Riebe, D. E. Granger, J. L. Clayton, J. G. King, and W.F. Megahan. 2001. Mountain erosion over 10-yr, 10-k.y., and 10-m.y. time scales. Geology 29: 591–594.
Kwak, T. J., W. E. Smith, E. N. Buttermore, P.
B. Cooney, and W. G. Cope. 2013. Fishery Population and Habitat assessment in Puerto Rico Streams: Phase 2 Final Report. Federal Aid in Sport Fish Restoration Project F-50 Final Report. Submitted to Marine Resources Division, Puerto Rico Department of Natural and Environmental Resources, San Juan.
Lee, D. C., J. R. Sedell, B. E. Rieman, R.F. Thurow, and J. E. Williams. 1997. Broadscale assessment of aquatic species and habitats. In An Assessment of Ecosystem Components in the Interior Columbia Basin and Portions of the Klamath and Great Basins, ed. T. M. Quigley, et al. (pp. 1058–1496). Portland, OR: US Department of Agriculture, Forest Service, PNW-GTR-405.
Lugo, A. E., and T. Heartsill Scalley. 2014. Research in the Luquillo Experimental Forest has advanced understanding of tropical forests and resolved management issues. In USDA Forest Service Experimental Forests and Ranges: Research for the Long Term, ed. D. C. Hayes, S. L. Stout, R. H. Crawford and A. P. Hoover (pp. 435–461). New York: Springer.
Martin, D. J., and L. E. Benda. 2001. Patterns of instream wood recruitment and transport at the watershed scale. Transactions of the American Fisheries Society 130: 940–958.
Martin, J., and P. Glick. 2008. A great wave rising: Solutions for Columbia and Snake River salmon in the age of global warming. Portland, OR: Light in the River Project.
Master, L. L., S. R. Flack, and B. A. Stein. 1998. Rivers of life: Critical watersheds for protecting freshwater biodiversity. Arlington, VA: The Nature Conservancy in cooperation with natural heritage programs and the Association for Biodiversity Information.
McGrath, A. 2014. Land of the free-flowing rivers: Administration of the 1968 Wild and Scenic Rivers Act on designated rivers in Oregon and Washington State. M.A. Thesis, University of Washington.
McManamay, R. A., D. J. Orth, C. A. Dolloff, and E. A. Frimpong. 2012. Regional frameworks applied to hydrology: Can landscape-based frameworks capture the hydrologic variability? River Research and Applications 28: 1325–1339.
McManamay, R. A., P. W. Bonsall, S. C. Hetrick, and B. T. Smith. 2013. Digital Mapping and Environmental Characterization of the National Wild and Scenic Rivers System. Oak Ridge National Laboratory Report ORNL/TM-2013/356.
Meehan, W. R., ed. 1991. Influences of Forest and Rangeland Management on Salmonid Fishes and Their Habitats. Bethesda, MD: American Fisheries Society Special Publication 19.
Meyer, G.A., and S. G. Wells. 1987. Fire-related sedimentation events on alluvial fans, Yellowstone National Park, USA. Journal of Sedimentary Research 67: 776–791.
Morais, P., and F. Daverat, eds. 2016. An Introduction to Fish Migration. Boca Raton, FL: CRC Press.
Mulholland P. J., and J. A. Watts. 1982. Transport of organic carbon to the oceans by rivers of North America: A synthesis of existing data. Tellus 34: 176–186.
NMFS (National Marine Fisheries Service). 2000. Biological opinion of the Federal Columbia River Power System, including the juvenile fish transportation program and the Bureau of Reclamation’s 31 dams including the entire Columbia Basin project. Retrieved from http://www.westcoast.fisheries.noaa.gov/fish_passage/fcrps_opinion/federal_ columbia_river_power_system.html
NRC (National Research Council). 1996. Upstream: Salmon and Society in the Pacific Northwest. Washington, DC: National Academy Press.
Neville, H. M., D. J. Isaak, J. B. Dunham, R. F. Thurow, and B. E. Rieman. 2006a. Fine-scale natal homing and localized movement as shaped by sex and spawning habitat in Chinook salmon: Insights from spatial autocorrelation analysis of individual genotypes. Molecular Ecology 15: 4589–4602.
Neville, H., J. B. Dunham, and M. Peacock. 2006b. Assessing connectivity in salmonid fishes with DNA microsatellite markers. In Connectivity Conservation, ed. K. R. Crooks and M. Sankayan (pp. 318–342). Cambridge, UK: Cambridge University Press.
Nilsson, C., C. A. Reidy, M. Dynesius, and C. Revenga. 2005. Fragmentation and flow regulation of the world’s large river systems. Science 308: 405–408.
Noss, R. F. 1991. Sustainability and wilderness. Conservation Biology 5: 120–122.
Ortíz-Zayas, J. R., and F. N. Scatena. 2004. Integrated water resources management in the Luquillo Mountains, Puerto Rico: An evolving process. Journal of Water Resources Development 20: 387–398.
Pahl-Wostl, C., D. Tabara, R. Bouwen, M. Craps, A. Dewulf, E. Mostert, D. Ridder, and T. Taillieu. 2008. The importance of social learning and culture for sustainable water management. Ecological Economics 64: 484–495.
Parrish, D. L., R. J. Behnke, S. R. Gephard, S. D. McCormick, and G. H. Reeves. 1998. Why aren’t there more Atlantic salmon (Salmo salar)? Canadian Journal of Fisheries and Aquatic Sciences 55: 281–287.
Pérez-Reyes, O., T. A. Crowl, and A. P. Covich. 2015. Effects of food supplies and water temperature on growth rates of two species of freshwater tropical shrimps. Freshwater Biology 60: 1514–1524.
Pierce, J. L., G. A. Meyer, and A. J. T. Jull. 2004. Fire-induced erosion and millennial-scale climate change in northern ponderosa pine forests. Nature 432: 87–90.
Pringle, C. M. 1997. Exploring how disturbance is transmitted upstream: Going against the flow. Journal of the North American Benthological Society 16: 425–438.
———. 2000. Threats to U.S. public lands from cumulative hydrologic alterations outside of their boundaries. Ecological Applications 10: 971–989.
———. 2001. Hydrologic connectivity and the management of biological reserves: A global perspective. Ecological Applications 11: 981–998.
Raymond, H. L. 1979. Effects of dams and impoundments on migrations of juvenile Chinook salmon and steelhead from the Snake River – 1966 to 1975. Transactions of the American Fisheries Society 108:505–529.
Reeves, G. H., L. E. Benda, K. M. Burnett, P. A. Bisson, and J. R. Sedell. 1995. A disturbance-based approach to maintaining and restoring freshwater habitats of evolutionarily significant units of anadromous salmonids in the Pacific Northwest. American Fisheries Society Symposium 17: 334–349.
Rieman, B. E., and D. J. Isaak. 2010. Climate Change, Aquatic Ecosystems, and Fishes in the Rocky Mountain West: Implications and Alternatives for Management. Gen. Tech. Rep. RMRS-GTR-250. Fort Collins, CO: US Department of Agriculture, Forest Service, Rocky Mountain Research Station.
Riley, K. E., J. L. Pierce, A. J. Hopkins, and G.B. Wright. 2011. Wildfires, debris flows, and climate: Using modern and ancient deposits to reconstruct Holocene sediment yields in central Idaho, EP21D-07. AGU Fall Meeting, San Francisco, CA, Dec. 5–9.
Rockström, J., and L. Karlberg. 2010. The quadruple squeeze: Defining the safe operating space for freshwater use to achieve a triply green revolution in the Anthropocene. AMBIO 39: 257–265.
Scatena, F. N., and S. L. Johnson. 2001. Instream-Flow Analysis for the Luquillo ExperimentalForest, Puerto Rico: Methods and Analysis. Gen. Tech. Rep. IITF-GTR-11. Rio Piedras, Puerto Rico: US Department of Agriculture, Forest Service, International Institute of Tropical Forestry.
Schlosser, I. J. 1991. Stream fish ecology: A landscape perspective. BioScience 41: 704–712.
Scott, W. B., and E. J. Crossman. 1973. Freshwater fishes of Canada. Fisheries Research Board of Canada Bulletin 184.
Seo, J. I., F. Nakamura, D. Nakano, H. Ichiyanagi, and K. W. Chun. 2008. Factors controlling the fluvial export of large woody debris, and its contribution to organic carbon budgets at watershed scales. Water Resources Research 44(4): 1-13.
Smith, J. W., and R. L. Moore. 2011. Perceptions of community benefits from two wild and scenic rivers. Environmental Management 47: 814–827.
Snieszko, S. F. 1974. The effects of environ- mental stress on outbreaks of infectious diseases of fishes. Journal of Fish Biology6: 197–208.
Strayer, D. L. 2010. Alien species in fresh waters: Ecological effects, interactions with other stressors, and prospects for the future. Freshwater Biology 55: 152–174.
Strayer, D. L., and D. Dudgeon. 2010. Freshwater biodiversity conservation: Recent progress and future challenges. Journal of the North American Benthological Society 29: 344–358.
Svenson, L. O. 2010. Fire and climate in a lodgepole forest of central Idaho: Annual, decadal, centennial, and millennial perspectives. MS thesis, Boise State University, Boise, ID.
Thurow, R. F. 2000. Dynamics of Chinook salmon populations within Idaho’s Frank Church wilderness: Implications for persistence. In Wilderness Science in a Time of Change Conference ed. S. F. McCool, D. N. Cole, W.
T. Borrie, and J. O’Loughlin (pp. 143–151). US Department of Agriculture, Forest Service. RMRS-P-15-VOL-3.
———. 2015. Salmon, landscapes, and functioning ecosystems: a wilderness lesson in mutual dependency. U.S. Department of Agriculture, Forest Service. Wag Tales-Newsletter of the Chief’s Wilderness Advisory Group. http://fsweb. wo.fs.fed.us/rhwr/wilderness/wag/ index_wag.html
Thurow, R. F., D. C. Lee, and B. E. Rieman. 1997. Distribution and status of seven native salmonids in the interior Columbia River Basin and portions of the Klamath River and Great Basins. North American Journal of Fisheries Management 17: 1094–1110.
———. 2000. Status and distribution of chinook salmon and steelhead in the interior Columbia River Basin and portions of the Klamath River Basin. In Sustainable Fisheries Management: Pacific Salmon, ed.E. Knudsen, C. Steward, D. MacDonald, J. Williams, and D. Reiser (pp. 133–160). Boca Raton, FL: CRC Press.
USFS (US Forest Service). 1993. Proposed Wild and Scenic Rivers, El Yunque National Forest. US Department of Agriculture, Forest Service. Retrieved from http://www.fs.usda.gov/detailfull/elyunque/home/%3Fcid%3Dfsbdev3_042977#wild_scenic.
———. 1998. Frank Church–River of No Return Wilderness programmatic and operational management plans. Draft Environmental
Impact Statement. Washington, DC: US Department of Agriculture, Forest Service.
Van Hyning, J. 1973. Factors affecting the abundance of fall Chinook salmon in the Columbia River. Research Reports of the Fish Commission of Oregon 4: 1–87.
Webster, D. A. 1982. Early history of the Atlantic salmon in New York. New York Fish and Game Journal 29: 26–44.
Westerling, A. L., H. G. Hidalgo, D. R. Cayan, and T. W. Swetnam. 2006. Warming and earlier spring increase western US forest wildfire activity. Science 313: 940–943.
Whitlock, C., C. E. Briles, M. C. Fernandez, and J. Gage. 2010. Holocene vegetation, fire and climate history of the Sawtooth Range, central Idaho, USA. Quaternary Research 75: 114–124.
Williams, J. D., M. L. Warren, K. S. Cummings, J. L. Harris, and R. J. Neves. 1993. Conser- vation status of freshwater mussels of the United States and Canada. Fisheries 18: 6–22.
Williams, J. E., R. N. Williams, R. F. Thurow, L. Elwell, D. P. Philipp, F. A. Harris, J. L. Kershner, P. J. Martinez, D. Miller, G. H. Reeves, C. A. Frissell, and J. R. Sedell. 2011. Native fish conservation areas: A vision for large-scale conservation of native fish communities. Fisheries 36: 267–277.
Williams, R. 2006. Return to the River: Restoring Salmon to the Columbia River. Boston, MA: Elsevier Academic Press.
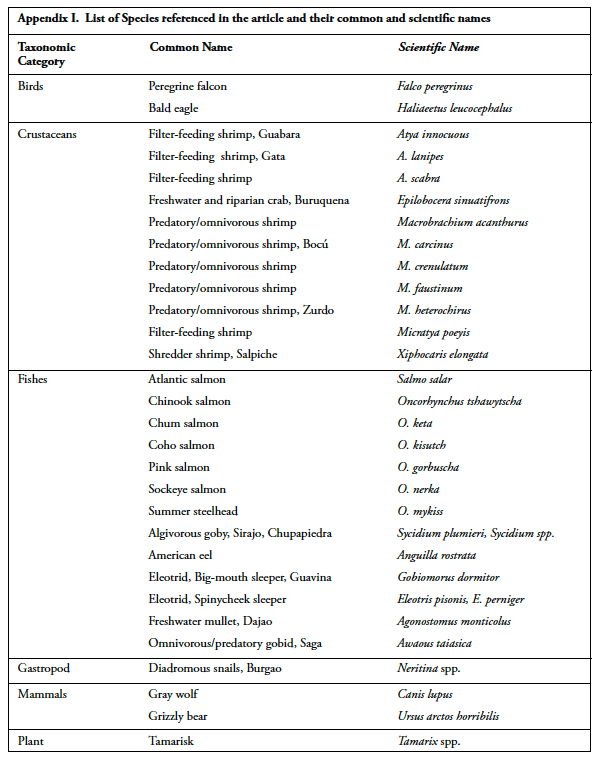